
Research & Initiatives
The Robichaux lab aims to discover how vision begins in our eyes on a molecular level. We apply these new molecular discoveries toward understanding the pathology of eye diseases that cause blindness. Our goal is to utilize these discoveries to learn how these devastating eye diseases can be treated and how vision can be rescued.
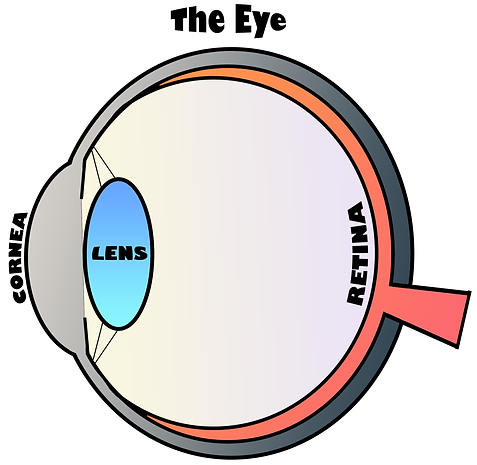
The retina is the neural tissue at the back of the eye. Photoreceptor neurons within the outer retina convert light that enters the eye into a bioelectric response. Photoreceptors, rods and cones, are specialized neurons with unique features that enable them to efficiently house the visual molecular signaling pathway, also known as the phototransduction pathway.
Opsin proteins are G-protein coupled receptors that absorb photons in photoreceptors and initiate visual signaling; they are essential for both vision and photoreceptor neuronal homeostasis. Rhodopsin is the opsin protein in rod photoreceptor neurons, and it is densely packaged into the distinctive layers of stacked membranes discs in a region of the photoreceptor known as the outer segment. The rod outer segment is a heavily-modified primary cilium with many essential cytoskeletal structures that are common to other kinds of cilia and flagella. Cone photoreceptors have their own specialized outer segment cilia.
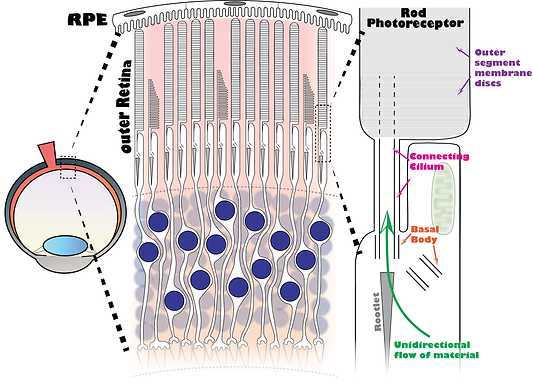
We are interested in discovering the mechanisms that regulate the cellular trafficking of rhodopsin and other proteins within rod photoreceptors. Retinal pigment epithelial (RPE) cells phagocytize the distal tips of rod outer segments on a daily basis. Therefore, a unidirectional flow of new material can be continuously trafficking through many unique ciliary structures in rod neurons by a thin connecting cilium that is only 300 nm in diameter. At the base of the cilia are a pair of centrioles that comprise the subcellular domain known as the basal body. Finally, a large cytoskeletal element known as the rootlet is linked onto the cilium.
In many eye diseases, retinal neurodegeneration is caused by the mistrafficking of rhodopsin in rods, that is, the inability or inhibition of rhodopsin molecules to be trafficked to the outer segment cilium. Such trafficking defects disrupt rod neuron homeostasis and lead to photoreceptor neuronal cell death. Because all rhodopsin molecules are trafficked to the outer cilium in rods, many cilia-associated proteins are integral to rod trafficking events. This ciliary integration, along with the unique structures and morphology of rod neurons, makes the rod photoreceptor cell biology unique and highly specialized subject of research.
Our interest in rhodopsin and the rod cilium is highly relevant to retinitis pigmentosa, which is a retinal neurodegenerative disease that affects 1 in 4,000 individuals in the United States (Hamel, 2006) and is the most prevalent human inherited retinal disease (Daiger et al., 2013). Retinitis pigmentosa is a progressive retinal degeneration that leads to gradual vision loss and blindness, and was named for the pigmentation that develops in the back of the eye of affected patients due to retina loss.

Picture of healthy human retina fundus
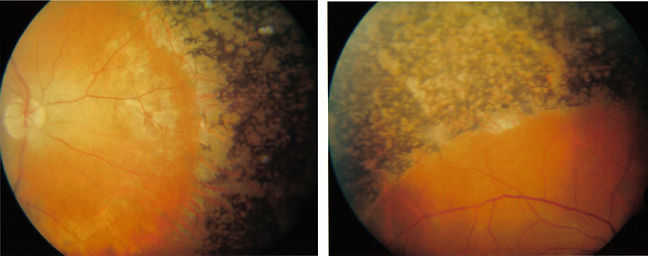
Picture of human eye with retinitis pigmentosa
Among the approximately 150 genes linked to retinitis pigmentosa, inherited mutations of rhodopsin are the leading cause of the autosomal dominant form of retinitis pigmentosa (adRP) (Athanasiou et al, 2018). One rhodopsin mutation alone, the P23H point mutation, accounts for 10% of all adRP cases (Sullivan et al., 2006). As such, the pathology of the P23H rhodopsin mutation has been studied throughout the field of vision research for decades.
In addition, disease mutations that affect cilia, known as ciliopathies, are syndromic conditions that typically lead to retinal neurodegeneration (Bujakowska et al., 2017). This is due to the essential function of the outer segment cilium in rods. Therefore, when this cilium is disrupted by a ciliopathy mutation, key cellular processes in rods, including rhodopsin trafficking, are disrupted, which leads to rod cell death, retinal degeneration, and blindness in human patients with ciliopathy mutations.
The Robichaux lab group is focused on studying rhodopsin trafficking in rod neurons on a subcellular level to understand how these critical cellular functions are integrated with the structures and dynamics of the rod cilium to collectively maintain rod cell homeostasis. Our approach is to use advanced forms of microscopy to visualize molecular events in single rod neurons of the mouse retina. Among these advanced microscopies is stochastic optical reconstruction microscopy (STORM), a form of super-resolution fluorescence microscopy. Dr. Robichaux led the research effort to use STORM to study subcellular events in the retinas for the first time (Robichaux et al., 2019).
Example of super-resolution microscopy. Data from Dr. Robichaux and colleagues (Tange et al., 2019)
The Robichaux lab at WVU will use STORM in combination with electron microscopy, expansion microscopy and other modes of super-resolution microscopy, along with protein biochemistry and tissue culture applications to study a range of exciting and relevant research topics in the fields of photoreceptor cell biology and eye disease.
Example of STORM super-resolution images of the endoplasmic reticulum in cultured cells; captured in the Robichaux Lab.
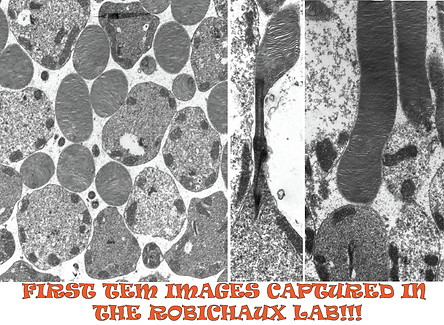
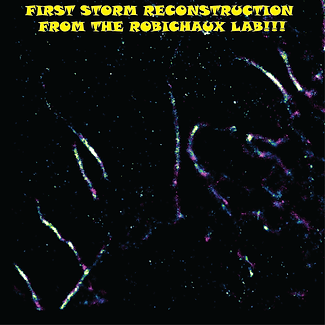
Research questions that we will test in the Robichaux lab:
-
How are rhodopsin molecules efficiently trafficked to the outer segment cilium in single rod neurons?
​
-
How do rod neurons adapt to the P23H rhodopsin retinitis pigmentosa mutation?
​
-
How do retinal gene therapy strategies affect diseased rob neurons on a subcellular level?
​
-
Do different retinal ciliopathies have a common molecular etiology?
​
-
What are new adaptations of super-resolution technology that enhances our ability to discover new molecular events in the retina?
References:
Hamel, C. (2006). Retinitis pigmentosa. Orphanet Journal of Rare Diseases, 1(1), 1–12. https://doi.org/10.1186/1750-1172-1-40
Daiger, S. P., Sullivan, L. S., & Bowne, S. J. (2013). Genes and mutations causing retinitis pigmentosa. Clinical Genetics, 84(2), 132–141. https://doi.org/10.1111/cge.12203
Athanasiou, D., Aguila, M., Bellingham, J., Li, W., McCulley, C., Reeves, P. J., & Cheetham, M. E. (2018). The molecular and cellular basis of rhodopsin retinitis pigmentosa reveals potential strategies for therapy. Progress in Retinal and Eye Research, 62, 1–23. https://doi.org/10.1016/j.preteyeres.2017.10.002
Sullivan, L. S., Bowne, S. J., Birch, D. G., Hughbanks-Wheaton, D., Heckenlively, J. R., Lewis, R. A., … Daiger, S. P. (2006). Prevalence of disease-causing mutations in families with autosomal dominant retinitis pigmentosa: A screen of known genes in 200 families. Investigative Ophthalmology and Visual Science, 47(7), 3052–3064. https://doi.org/10.1167/iovs.05-1443
Bujakowska, K. M., Liu, Q., & Pierce, E. A. (2017). Photoreceptor cilia and retinal ciliopathies. Cold Spring Harbor Perspectives in Biology, 9(10). https://doi.org/10.1101/cshperspect.a028274
Robichaux, M. A., Potter, V. L., Zhang, Z., He, F., Liu, J., Schmid, M. F., & Wensel, T. G. (2019). Defining the layers of a sensory cilium with STORM and cryoelectron nanoscopy. Proceedings of the National Academy of Sciences, 116(47), 23562–23572. https://doi.org/10.1073/pnas.1902003116
​
Tang, J., Robichaux, M. A., Wu, K.-L., Pei, J., Nguyen, N. T., Zhou, Y., … Xiao, H. (2019). Single-Atom Fluorescence Switch: A General Approach toward Visible-Light-Activated Dyes for Biological Imaging. Journal of the American Chemical Society, 141(37), 14699–14706. research-article. https://doi.org/10.1021/jacs.9b06237
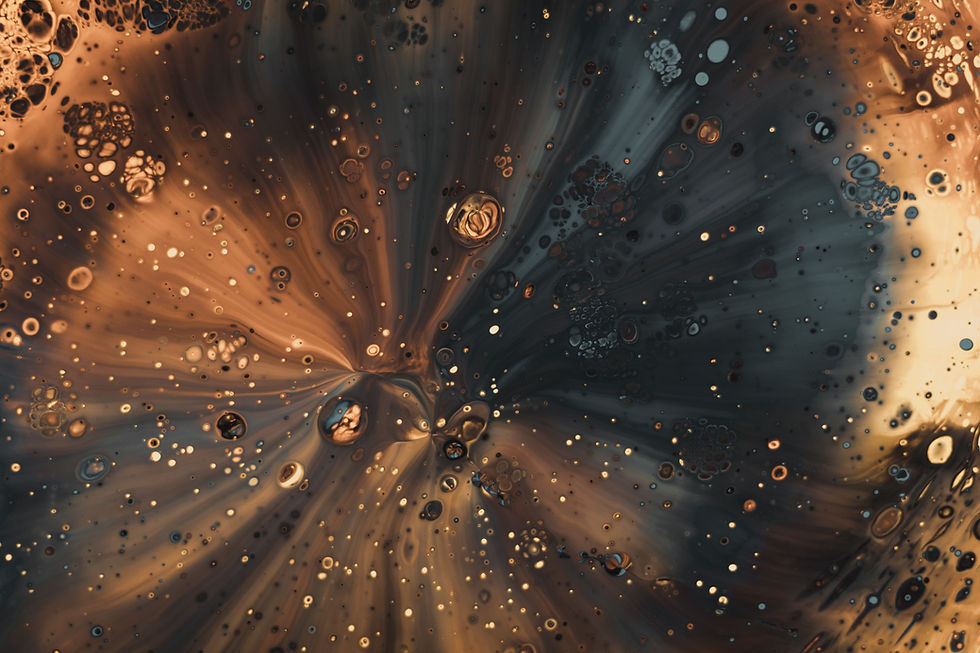